Carbon starvation during drought-induced tree mortality – are we chasing a myth?
Abstract
Drought-induced tree mortality has received much attention in the recent past. McDowell et al.’s (2008) hydraulic framework links tree hydraulics with carbon dynamics and proposes two non-exclusive mortality mechanisms: carbon starvation (CS) and hydraulic failure (HF). CS is often referred to as the (partial) depletion of non-structural carbohydrates (NSC) in response to stomatal closure, reduced C assimilation and sustained C storage dependency during longer droughts. HF describes a lethal level of xylem dysfunction from runaway embolism during severe droughts. While HF can be readily inferred from the percentage loss of conductivity in vascular tissues at the time of death, CS is much more difficult to assess.
Starvation is usually defined as a lack of food leading to suffering or death. In plants photosynthetic sugars play many functional roles, not only as a source of catabolic energy. For example, sugars are important for osmotic regulation of cell pressure and recent studies suggest a potential link between xylem parenchyma sugars and embolism repair following drought. Hence, carbon limitation could have a direct impact on tree hydraulics and HF; however, empirical evidence for such a mechanism is still inconclusive.
Although HF appears to be predominant during drought mortality, our limited understanding of the roles of NSC in hydraulic function precludes any premature refutation of CS as a mechanism in drought-induced tree mortality.
Plant carbon dynamics during drought - no water, no carbon
Recent observations of increased drought-induced tree and forest mortality across the globe (e.g.,Allen et al., 2010; Settele et al., 2014) have incited a wave of investigations on the underlying causal mechanisms (Hartmann et al., 2015). Even under non drought conditions, plants have to spend hundreds of molecules of water for each molecule of carbon dioxide they assimilate (Taiz & Zeiger, 2002). In vascular plants stomata play a critical role in regulating this exchange. When soil water availability is reduced, root signaling via phytohormones cause stomatal closure as a means to decrease water loss via transpiration (Brodribb & McAdam, 2011). However, stomatal closure also reduces CO2 diffusion into the leaf and hence carbon assimilation. It is therefore no surprise that the plant carbon balance is tightly coupled with its water balance.
McDowell et al.’s (2008) hydraulic framework employed this relationship to explain how drought severity and drought duration, in interaction with a species’ stomatal behavior and its capacity to maintain a minimum water potential, may lead to either hydraulic failure (irreversible xylem dysfunction, HF) or carbon starvation (CS), i.e. insufficient carbon supply to meet a plant’s demand for both osmotic and metabolic functioning (McDowell, 2011). An anisohydric (aniso = unequal, hydric = relating to moisture) species, i.e. a species that can maintain very low water potentials during drought, would also experience very low xylem water tension with the threat of cavitation and run-away embolism during a severe drought – and hence may die from HF. On the other hand, more conservative (isohydric) species that cannot maintain very low water potentials during drought would face sustained reductions in carbon assimilation during longer droughts and may then have to rely on stored carbohydrates (or other stored carbon compounds) until these are depleted – and would die from CS.
Carbon starvation - what exactly does that mean?
Merriam-Webster® online dictionary defines starvation as “suffering or death caused by having nothing to eat or not enough to eat: the condition of someone who is starving”. Plants produce their own food during photosynthesis in form of sugars like glucose. While drought reduces stomatal conductivity and hence glucose production, plants usually store large amounts of carbon compounds and hence do not suffer immediately after the onset of drought. On the other hand, glucose and other non-structural carbon compounds (NSC) derived from glucose play many roles in plant functioning, not only as sources of catabolic energy. Besides their role as substrates for plant defense compounds, carbohydrates are the building blocks for structural biomass and serve also as signaling substances for the genetic regulation of metabolic processes like photosynthesis or respiration (Koch, 1996). Also, sugars are important for controlling osmotic cell pressure to prevent tissue desiccation and to maintain physiological functioning under decreasing water availability (Morgan, 1984). Hence, severe reductions in carbohydrates not only influence plant catabolism but plant functioning in general and, in particular, the hydraulic functioning.
There are many potential interactions relating severe reductions in NSC (i.e. the lack of ‘free’ carbon compounds) to the plant water balance, for example xylem and root growth, osmotic regulation or xylem embolism repair. Observations of xylem recovery from large loss of hydraulic conductivity after drought have initially been explained by basal area growth, i.e. replacement of embolized vessels with new functional ones (Brodribb et al., 2010). Also, plants may overcome water deficits by allocating carbon to root growth to maintain a functional equilibrium of the root:shoot ratio under drought conditions (Poorter & Nagel, 2000) or to mycorrhizal hyphae allowing exploring greater soil volumes (Lehto & Zwiazek, 2011). Moreover, recent theoretical advances show that embolism may be repaired with water inflow from surrounding functional vessels and this inflow would be driven by an osmotic gradient established via importing low molecular-weight sugars into embolized conduits (Brodersen et al., 2010; Nardini et al., 2011). However, methodological artefacts during conductivity measurements may yield spurious evidence for embolism repair (Sperry, 2013; Wheeler et al., 2013) and the prevalence of xylem refilling in trees may be greatly overestimated (Cochard & Delzon, 2013; Delzon & Cochard, 2014). On the other hand, Thorsten Knipfer presented during the 2015 Xylem International Meeting in Bordeaux experimental results showing that embolism repair in grapevines, observed via X-ray microtomography, was related to the presence of carbohydrates in xylem parenchyma cells. While such results have not yet been shown for tree species (Knipfer, T, personal communication), evidence from a variety of drought manipulations in potted trees suggests a linkage between xylem vulnerability and xylem carbohydrate content (Adams et al., in preparation) and is hence supportive of a similar mechanism acting in trees. The fact that trees store substantial amounts of NSC in parenchyma cell in the vicinity of xylem vessels (Plavcová & Jansen, 2015) also suggests a potential functional role for the maintenance of water transport. However, given the lack of empirical support for a functional linkage between xylem carbohydrates and hydraulic function such suggestions are still rather speculative (Fig. 1).
Carbon starvation - does it exist beyond theory?
Carbon dynamics during starvation has so far been investigated only in cell cultures, excised tissues or shaded herbaceous plants (e.g., Journet et al., 1986; Brouquisse et al., 1991; Dieuaide-Noubhani et al., 1997; Devaux et al., 2003; Tcherkez et al., 2003; Morkunas et al., 2012). Experimental studies on drought-induced tree mortality are rather inconclusive with respect to carbon storage changes. Carbohydrate concentrations sometimes decline (e.g., Galiano et al., 2011; Galvez et al., 2013; Poyatos et al., 2013) and sometimes increase during lethal drought (e.g., Galvez et al., 2011; Anderegg et al., 2012; Hartmann et al., 2013b) while HF (measured as substantial loss of branch conductivity) has been observed in many other studies (e.g., Anderegg & Anderegg, 2013; Duan et al., 2013; Mitchell et al., 2013). Moreover, very few investigations have been carried out that were able to partition drought effects on carbon assimilation from drought effects on a tree’s capacity to translocate stored carbon from sources to sinks (McDowell & Sevanto, 2010). Declining plant hydration during drought negatively affects phloem transport either via phloem viscosity buildup or reduced phloem cell turgor (Sevanto, 2014; Mencuccini et al., 2015) and impeded carbon translocation may be yet another causal mechanism of drought-induced tree mortality (Sala et al., 2010). Only two studies have addressed this mechanism explicitly and showed that phloem transport failure may indeed be responsible for drought mortality (Hartmann et al., 2013a; Sevanto et al., 2014). Impeded carbon transport across plant tissues may be causing different mechanisms to operate in different plant tissues and may cause HF to occur in above-ground tissues while belowground tissues die from CS (Hartmann et al., 2013a; Hartmann et al., 2013b). How such processes interact with other changes in environmental conditions, such as elevated temperature, has so far only been investigated under controlled experimental conditions. Such studies show that elevated temperatures speed up plant carbon expenditure by increasing respiration rates (Adams et al., 2009) and push plants faster into a negative carbon balance (Zhao et al., 2013) and could – likely – cause or hasten CS. However, elevated temperature also increases water vapor pressure deficit and hence decreases xylem water potential thereby yielding a greater risk of HF. Such interactions may explain why experimental drought manipulations of potted plants indicated a prevalence of HF as mortality mechanism while reduced carbohydrate concentrations were common but not universal with mortality (Adams, in preparation).
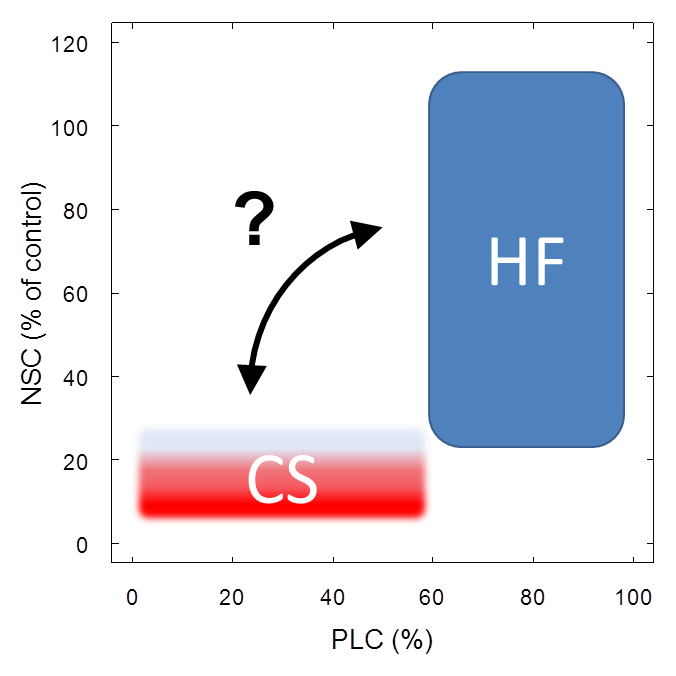
Figure 1: Working definitions for carbon starvation and hydraulic failure. Carbon starvation is defined as ‘depletion’ (compared to control trees) of carbohydrate pools. Minimum levels of carbohydrates may vary across plant types and even across plant tissues but are currently unknown (indicated by fuzzy shape). In contrast, thresholds for hydraulic failure (loss of conductivity) also depend on plant type and other factors but the range of thresholds is comparably well defined (indicated by clear shape). Interactions between minimum carbohydrate levels and hydraulic function, like carbohydrate-mediated embolism repair (arrow), are still not well understood and require further investigations.
Carbon starvation - are we chasing a myth?
There are still many unresolved questions with respect to tree carbon metabolism during drought and simple measurements of NSC concentrations may not be very insightful with respect to the tree carbon balance (Ryan, 2011). Carbon starvation can clearly not be defined as a complete depletion of NSC pools, as starvation in mammals occurs before blood sugar levels are down to zero (Hoch, 2015). Also, some NSC may be sequestered in woody tissues and are likely not be available for plant metabolism (Sala et al., 2012). While thresholds for HF are well defined and have been reported for both conifers (e.g., Brodribb & Cochard, 2009) and angiosperms (e.g., Barigah et al., 2013b; Urli et al., 2013) minimum thresholds of NSC concentrations required for survival are currently unknown and this prevents defining useful working definitions for CS (Fig. 1). Methodological issues in measuring carbohydrates in plant tissues still prevent us from accurately determining absolute carbohydrate concentrations (Quentin et al., 2015) and trees may shift carbon catabolism away from carbohydrates towards lipids during carbon limitation (Fischer et al., 2015; Hanf et al., 2015). Hence carbohydrate concentration, often assessed in studies on tree responses to drought, may be elusive and misleading.
Future research should focus on the role of NSC, including lipids, in key plant tissues like meristems that are essential for regrowth after disturbance and for plant survival (Barigah et al., 2013a). As long as the functional roles of NSC in such key tissues are not clearly identified and addressed in focused studies, any attempt to refute the existence of CS during drought-induced tree mortality will be premature and scientifically unsound.
Acknowledgements : this opinion paper is based on a keynote talk I presented at the Xylem International meeting, held in Bordeaux on Sept 7-9, 2015. I thank Hervé Cochard, Sylvain Delzon and José Torrez-Ruiz for inviting me to the meeting and all other participants for insightful and interesting discussions.
References
- Adams HD, Guardiola-Claramonte M, Barron-Gafford GA, Villegas JC, Breshears DD, Zou CB, Troch PA, Huxman TE. 2009. Temperature sensitivity of drought-induced tree mortality portends increased regional die-off under global-change-type drought. Proceedings of the National Academy of Sciences 106: 7063-7066. doi:10.1073/pnas.0901438106
- Allen CD, Macalady AK, Chenchouni H, Bachelet D, McDowell N, Vennetier M, Kitzberger T, Rigling A, Breshears DD, Hogg EH, et al. 2010. A global overview of drought and heat-induced tree mortality reveals emerging climate change risks for forests. Forest Ecology and Management 259: 660-684. doi:10.1016/j.foreco.2009.09.001
- Anderegg WRL, Anderegg LDL. 2013. Hydraulic and carbohydrate changes in experimental drought-induced mortality of saplings in two conifer species. Tree Physiology 33: 252-260. doi:10.1093/treephys/tpt016
- Anderegg WRL, Berry JA, Smith DD, Sperry JS, Anderegg LDL, Field CB. 2012. The roles of hydraulic and carbon stress in a widespread climate-induced forest die-off. Proceedings of the Nat. Academy of Sciences 109: 233-237. doi:10.1073/pnas.1107891109
- Barigah TS, Bonhomme M, Lopez D, Traore A, Douris M, Venisse J-S, Cochard H, Badel E. 2013a. Modulation of bud survival in Populus nigra sprouts in response to water stress-induced embolism. Tree Physiology 33: 261-274. doi:10.1093/treephys/tpt002
- Barigah TS, Charrier O, Douris M, Bonhomme M, Herbette S, Améglio T, Fichot R, Brignolas F, Cochard H. 2013b. Water stress-induced xylem hydraulic failure is a causal factor of tree mortality in beech and poplar. Annals of Botany 112:1431-1437. doi:10.1093/aob/mct204
- Brodersen CR, McElrone AJ, Choat B, Matthews MA, Shackel KA. 2010. The Dynamics of Embolism Repair in Xylem: In Vivo Visualizations Using High-Resolution Computed Tomography. Plant Physiology 154: 1088-1095. doi:10.1104/pp.110.162396
- Brodribb TJ, Bowman DJMS, Nichols S, Delzon S, Burlett R. 2010. Xylem function and growth rate interact to determine recovery rates after exposure to extreme water deficit. New Phytologist 188: 533-542. doi:10.1111/j.1469-8137.2010.03393.x
- Brodribb TJ, Cochard H. 2009. Hydraulic Failure Defines the Recovery and Point of Death in Water-Stressed Conifers. Plant Physiology 149: 575-584. doi:10.1104/pp.108.129783
- Brodribb TJ, McAdam SAM. 2011. Passive Origins of Stomatal Control in Vascular Plants. Science 331: 582-585. doi:10.1126/science.1197985
- Brouquisse R, James F, Raymond P, Pradet A. 1991. Study of glucose starvation in excised maize root tips. Plant Physiology 96: 619-626. doi:10.1104/pp.96.2.619
- Cochard H, Delzon S. 2013. Hydraulic failure and repair are not routine in trees. Annals of Forest Science 70: 659-661. doi:10.1007/s13595-013-0317-5
- Delzon S, Cochard H. 2014. Recent advances in tree hydraulics highlight the ecological significance of the hydraulic safety margin. New Phytologist 203: 355-358. doi:10.1111/nph.12798
- Devaux C, Baldet P, Joubès J, Dieuaide‐Noubhani M, Just D, Chevalier C, Raymond P. 2003. Physiological, biochemical and molecular analysis of sugar‐starvation responses in tomato roots. Journal of Experimental Botany 54: 1143-1151. doi:10.1093/jxb/erg113
- Dieuaide-Noubhani M, Canioni P, Raymond P. 1997. Sugar-starvation-induced changes of carbon metabolism in excised maize root tips. Plant Physiology 115: 1505-1513.
- Duan H, Amthor JS, Duursma RA, O'Grady AP, Choat B, Tissue DT. 2013. Carbon dynamics of eucalypt seedlings exposed to progressive drought in elevated [CO2] and elevated temperature. Tree Physiology 33: 779-792. doi:10.1093/treephys/tpt061
- Fischer S, Hanf S, Frosch T, Gleixner G, Popp J, Trumbore S, Hartmann H. 2015. Pinus sylvestris switches respiration substrates under shading but not during drought. New Phytologist 207: 542-550. doi:10.1111/nph.13452
- Galiano L, Martínez-Vilalta J, Lloret F. 2011. Carbon reserves and canopy defoliation determine the recovery of Scots pine 4 yr after a drought episode. New Phytologist 190: 750-759. doi:10.1111/j.1469-8137.2010.03628.x
- Galvez DA, Landhausser SM, Tyree MT. 2013. Low root reserve accumulation during drought may lead to winter mortality in poplar seedlings. New Phytologist 198: 139-148. doi:10.1111/nph.12129
- Galvez DA, Landhäusser SM, Tyree MT. 2011. Root carbon reserve dynamics in aspen seedlings: does simulated drought induce reserve limitation? Tree Physiology 31: 250-257. doi:10.1093/treephys/tpr012
- Hanf S, Fischer S, Hartmann H, Keiner R, Trumbore S, Popp J, Frosch T. 2015. Online investigation of respiratory quotients in Pinus sylvestris and Picea abies during drought and shading by means of cavity-enhanced Raman multi-gas spectrometry. Analyst 140: 4473-4481. doi:10.1039/C5AN00402K
- Hartmann H, Adams HD, Anderegg WR, Jansen S, Zeppel MJ. 2015. Research frontiers in drought-induced tree mortality: crossing scales and disciplines. New Phytologist 205: 965-969. doi:10.1111/nph.13246
- Hartmann H, Ziegler W, Kolle O, Trumbore S. 2013a. Thirst beats hunger – declining hydration during drought prevents carbon starvation in Norway spruce saplings. New Phytologist 200: 340-349. doi:10.1111/nph.12331
- Hartmann H, Ziegler W, Trumbore S. 2013b. Lethal drought leads to reduction in nonstructural carbohydrates in Norway spruce tree roots but not in the canopy. Functional Ecology 27: 413-427. doi:10.1111/1365-2435.12046
- Hoch G 2015. Carbon Reserves as Indicators for Carbon Limitation in Trees. In: Lüttge U, Beyschlag W eds. Progress in Botany: Springer International Publishing, 321-346. doi:10.1007/978-3-319-08807-5_13
- Journet EP, Bligny R, Douce R. 1986. Biochemical changes during sucrose deprivation in higher plant cells. Journal of Biological Chemistry 261: 3193-3199.
- Koch KE. 1996. Carbohydrate-modulated gene expression in plants. Annual Review of Plant Physiology and Plant Molecular Biology 47: 509-540. doi:10.1146/annurev.arplant.47.1.509
- Lehto T, Zwiazek J. 2011. Ectomycorrhizas and water relations of trees: a review. Mycorrhiza 21: 71-90. doi:10.1007/s00572-010-0348-9
- McDowell N, Pockman WT, Allen CD, Breshears DD, Cobb N, Kolb T, Plaut J, Sperry J, West A, Williams DG, et al. 2008. Mechanisms of plant survival and mortality during drought: why do some plants survive while others succumb to drought? New Phytologist 178: 719-739. doi:10.1111/j.1469-8137.2008.02436.x
- McDowell NG. 2011. Mechanisms linking drought, hydraulics, carbon metabolism, and vegetation mortality. Plant Physiology 155: 1051-1059. doi:10.1104/pp.110.170704
- McDowell NG, Sevanto S. 2010. The mechanisms of carbon starvation: how, when, or does it even occur at all? New Phytologist 186: 264-266. doi:10.1111/j.1469-8137.2010.03232.x
- Mencuccini M, Minunno F, Salmon Y, Martínez-Vilalta J, Hölttä T. 2015. Coordination of physiological traits involved in drought-induced mortality of woody plants. New Phytologist 208: 396-409. doi:10.1111/nph.13461
- Mitchell PJ, O'Grady AP, Tissue DT, White DA, Ottenschlaeger ML, Pinkard EA. 2013. Drought response strategies define the relative contributions of hydraulic dysfunction and carbohydrate depletion during tree mortality. New Phytologist 197: 862-872. doi:10.1111/nph.12064
- Morgan JM. 1984. Osmoregulation and water stress in higher plants. Annual Review of Plant Physiol 35: 299-319. doi:10.1146/annurev.pp.35.060184.001503
- Morkunas I, Formela M, Borek S, Ratajczak L 2012. Plant responses to sugar starvation. Carbohydrates - Comprehensive Studies on Glycobiology and Glycotechnology, 409-438.
- Nardini A, Lo Gullo MA, Salleo S. 2011. Refilling embolized xylem conduits: Is it a matter of phloem unloading? Plant Science 180: 604-611. doi:10.1016/j.plantsci.2010.12.011
- Plavcová L, Jansen S 2015. The role of xylem parenchyma in the storage and utilization of nonstructural carbohydrates. In: Hacke U ed. Functional and Ecological Xylem Anatomy: Springer Intern. Publishing, 209-234. doi:10.1007/978-3-319-15783-2_8
- Poorter H, Nagel O. 2000. The role of biomass allocation in the growth response of plants to different levels of light, CO2, nutrients and water: a quantitative review. Functional Plant Biology 27: 1191-1191. doi:10.1071/PP99173
- Poyatos R, Aguade D, Galiano L, Mencuccini M, Martinez-Vilalta J. 2013. Drought-induced defoliation and long periods of near-zero gas exchange play a key role in accentuating metabolic decline of Scots pine. New Phytologist 200: 388-401. doi:10.1111/nph.12278
- Quentin AG, Pinkard EA, Ryan MG, Tissue DT, Baggett LS, Adams HD, Maillard P, Marchand J, Landhausser SM, Lacointe A, et al. 2015. Non-structural carbohydrates in woody plants compared among laboratories. Tree Physiology: tpv073. doi:10.1093/treephys/tpv073
- Ryan MG. 2011. Tree responses to drought. Tree Physiology 31: 237-239. doi:10.1093/treephys/tpr022
- Sala A, Piper F, Hoch G. 2010. Physiological mechanisms of drought-induced tree mortality are far from being resolved. New Phytologist 186: 274-281. doi:10.1111/j.1469-8137.2009.03167.x
- Sala A, Woodruff DR, Meinzer FC. 2012. Carbon dynamics in trees: feast or famine? Tree Physiology 32: 764-775. doi:10.1093/treephys/tpr143
- Settele J, Scholes R, Betts R, Bunn SE, Leadley P, Nepstad D, Overpeck JT, Taboada MA 2014. Terrestrial and inland water systems. In: Field CB et al eds. Climate Change 2014: Impacts, Adaptation, and Vulnerability. Part A: Global and Sectoral Aspects. Cambridge, United Kingdom and New York, USA Cambridge Univ Press, 271-359.
- Sevanto S. 2014. Phloem transport and drought. Journal of Experimental Botany 65: 1751-1759. doi:10.1093/jxb/ert467
- Sevanto S, McDowell NG, Dickman LT, Pangle R, Pockman WT. 2014. How do trees die? A test of the hydraulic failure and carbon starvation hypotheses. Plant, Cell & Environment 37: 153-161. doi:10.1111/pce.12141
- Sperry J. 2013. Cutting-edge research or cutting-edge artefact? An overdue control experiment complicates the xylem refilling story. Plant, Cell & Environment 36: 1916-1918. doi:10.1111/pce.12148
- Taiz L, Zeiger E. 2002. Plant physiology. Sunderland, Mass: Sinauer.
- Tcherkez G, Nogues S, Bleton J, Cornic G, Badeck F, Ghashghaie J. 2003. Metabolic origin of carbon isotope composition of leaf dark-respired CO2 in French bean. Plant Physiology 131: 237-244. doi:10.1104/pp.013078
- Urli M, Porte AJ, Cochard H, Guengant Y, Burlett R, Delzon S. 2013. Xylem embolism threshold for catastrophic hydraulic failure in angiosperm trees. Tree Physiology 33: 672-683. doi:10.1093/treephys/tpt030
- Wheeler JK, Huggett BA, Tofte AN, Rockwell FE, Holbrook NM. 2013. Cutting xylem under tension or supersaturated with gas can generate PLC and the appearance of rapid recovery from embolism. Plant, Cell & Environment 36: 1938-1949. doi:10.1111/pce.12139
- Zhao J, Hartmann H, Trumbore S, Ziegler W, Zhang Y. 2013. High temperature causes negative whole-plant carbon balance under mild drought. New Phytologist 200: 330-339. doi:10.1111/nph.12400
Attachments
No supporting information for this articleArticle statistics
Views: 5053
Downloads
PDF: 735